Abstract
Microbial lung infections are the major cause of morbidity and mortality in the hereditary metabolic disorder cystic fibrosis, yet the molecular mechanisms leading from the mutation of cystic fibrosis transmembrane conductance regulator (CFTR) to lung infection are still unclear. Here, we show that ceramide age-dependently accumulates in the respiratory tract of uninfected Cftr-deficient mice owing to an alkalinization of intracellular vesicles in Cftr-deficient cells. This change in pH results in an imbalance between acid sphingomyelinase (Asm) cleavage of sphingomyelin to ceramide and acid ceramidase consumption of ceramide, resulting in the higher levels of ceramide. The accumulation of ceramide causes Cftr-deficient mice to suffer from constitutive age-dependent pulmonary inflammation, death of respiratory epithelial cells, deposits of DNA in bronchi and high susceptibility to severe Pseudomonas aeruginosa infections. Partial genetic deficiency of Asm in Cftr−/−Smpd1+/− mice or pharmacological treatment of Cftr-deficient mice with the Asm blocker amitriptyline normalizes pulmonary ceramide and prevents all pathological findings, including susceptibility to infection. These data suggest inhibition of Asm as a new treatment strategy for cystic fibrosis.
This is a preview of subscription content, access via your institution
Access options
Subscription info for Japanese customers
We have a dedicated website for our Japanese customers. Please go to natureasia.com to subscribe to this journal.
Buy this article
- Purchase on SpringerLink
- Instant access to full article PDF
Prices may be subject to local taxes which are calculated during checkout
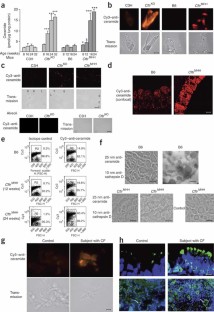
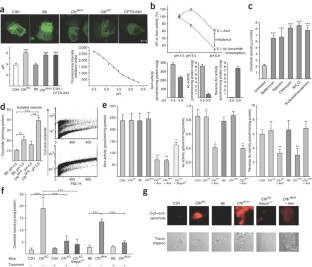
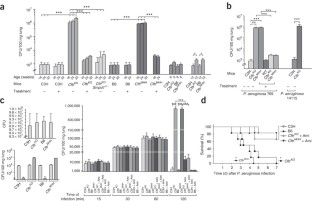
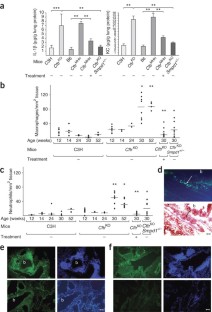
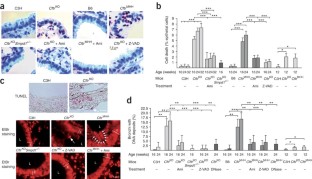
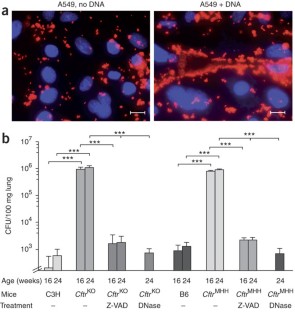
Similar content being viewed by others
References
Rommens, J.M. et al. Identification of the cystic fibrosis gene: chromosome walking and jumping. Science 245, 1059–1065 (1989).
Riordan, J.R. et al. Identification of the cystic fibrosis gene: cloning and characterization of complementary DNA. Science 245, 1066–1073 (1989).
Kerem, B. et al. Identification of the cystic fibrosis gene: genetic analysis. Science 245, 1073–1080 (1989).
Davis, P.B., Drumm, M. & Konstan, M.W. Cystic fibrosis. Am. J. Respir. Crit. Care Med. 154, 1229–1256 (1996).
Engelhardt, J.F. et al. Submucosal glands are the predominant site of CFTR expression in the human bronchus. Nat. Genet. 2, 240–248 (1992).
Kreda, S.M. et al. Characterization of wild–type and deltaF508 cystic fibrosis transmembrane regulator in human respiratory epithelia. Mol. Biol. Cell 16, 2154–2167 (2005).
Weber, A.J., Soong, G., Bryan, R., Saba, S. & Prince, A. Activation of NF-
κ B in airway epithelial cells is dependent on CFTR trafficking and Cl− channel function. Am. J. Physiol. Lung Cell. Mol. Physiol. 281, L71–L78 (2001).Joseph, T., Look, D. & Ferkol, T. NF–
κ B activation and sustained IL-8 gene expression in primary cultures of cystic fibrosis airway epithelial cells stimulated with Pseudomonas aeruginosa. Am. J. Physiol. Lung Cell. Mol. Physiol. 288, L471–L479 (2005).Zahm, J.M. et al. Early alterations in airway mucociliary clearance and inflammation of the lamina propria in CF mice. Am. J. Physiol. 272, C853–C859 (1997).
Tirouvanziam, R. et al. Inflammation and infection in naive human cystic fibrosis airway grafts. Am. J. Respir. Cell Mol. Biol. 23, 121–127 (2000).
Borst, P. & Elferink, R.O. Mammalian ABC transporters in health and disease. Annu. Rev. Biochem. 71, 537–592 (2002).
Boujaoude, L.C. et al. Cystic fibrosis transmembrane regulator regulates uptake of sphingoid base phosphates and lysophosphatidic acid: modulation of cellular activity of sphingosine-1-phosphate. J. Biol. Chem. 276, 35258–35264 (2001).
Barasch, J. et al. Defective acidification of intracellular organelles in cystic fibrosis. Nature 352, 70–73 (1991).
Di, A. et al. CFTR regulates phagosome acidification in macrophages and alters bactericidal activity. Nat. Cell Biol. 8, 933–944 (2006).
Dbaibo, G.S. & Hannun, Y.A. Signal transduction and the regulation of apoptosis: roles of ceramide. Apoptosis 3, 317–334 (1998).
Gulbins, E. & Kolesnick, R. Raft ceramide in molecular medicine. Oncogene 22, 7070–7077 (2003).
Wiegmann, K., Schutze, S., Machleidt, T., Witte, D. & Kronke, M. Functional dichotomy of neutral and acidic sphingomyelinases in tumor necrosis factor signaling. Cell 78, 1005–1015 (1994).
Grassme, H. et al. Host defense against Pseudomonas aeruginosa requires ceramide-rich membrane rafts. Nat. Med. 9, 322–330 (2003).
He, X. et al. Purification and characterization of recombinant, human acid ceramidase. J. Biol. Chem. 278, 32978–32986 (2003).
Spence, M.W., Byers, D.M., Palmer, F.B.S.C. & Cook, H.W. A new Zn2+-stimulated sphingomyelinase in fetal bovine serum. J. Biol. Chem. 264, 5358–5363 (1989).
Quintern, L.E. et al. Isolation of cDNA clones encoding human acid sphingomyelinase: occurrence of alternatively processed transcripts. EMBO J. 8, 2469–2473 (1989).
Futerman, A.H. & Riezman, H. The ins and outs of sphingolipid synthesis. Trends Cell Biol. 15, 312–318 (2005).
Menaldino, D.S. et al. Sphingoid bases and de novo ceramide synthesis: enzymes involved, pharmacology and mechanisms of action. Pharmacol. Res. 47, 373–381 (2003).
Hurwitz, R., Ferlinz, K. & Sandhoff, K. The tricyclic antidepressant desipramine causes proteolytic degradation of lysosomal sphingomyelinase in human fibroblasts. Biol. Chem. Hoppe Seyler 375, 447–450 (1994).
Elojeimy, S. et al. New insights on the use of desipramine as an inhibitor for acid ceramidase. FEBS Lett. 580, 4751–4756 (2006).
Coleman, F.T. et al. Hypersusceptibility of cystic fibrosis mice to chronic Pseudomonas aeruginosa oropharyngeal colonization and lung infection. Proc. Natl. Acad. Sci. USA 100, 1949–1954 (2003).
Pier, G.B. et al. Role of mutant CFTR in hypersusceptibility of cystic fibrosis subjects to lung infections. Science 271, 64–67 (1996).
Rodriguez, I., Matsuura, K., Ody, C., Nagata, S. & Vassalli, P. Systemic injection of a tripeptide inhibits the intracellular activation of CPP32-like proteases in vivo and fully protects mice against Fas-mediated fulminant liver destruction and death. J. Exp. Med. 184, 2067–2072 (1996).
Guilbault, C. et al. Fenretinide corrects newly found ceramide deficiency in cystic fibrosis. Am. J. Respir. Cell Mol. Biol. 38, 47–56 (2008).
Bhuvaneswaran, C., Venkatesan, S. & Mitropoulos, K.A. Lysosomal accumulation of cholesterol and sphingomyelin: evidence for inhibition of acid sphingomyelinase. Eur. J. Cell Biol. 73, 98–106 (1985).
Mol, M.J., Erkelens, D.W., Leuven, J.A., Schouten, J.A. & Stalenhoef, A.F. Effects of synvinolin (MK-733) on plasma lipids in familial hypercholesterolaemia. Lancet 328, 936–939 (1986).
Kolesnick, R.N., Goni, F.M. & Alonso, A. Compartmentalization of ceramide signaling: physical foundations and biological effects. J. Cell. Physiol. 184, 285–300 (2000).
Kasper, D. et al. Loss of the chloride channel ClC-7 leads to lysosomal storage disease and neurodegeneration. EMBO J. 24, 1079–1091 (2005).
Hara–Chikuma, M. et al. ClC-3 chloride channels facilitate endosomal acidification and chloride accumulation. J. Biol. Chem. 280, 1241–1247 (2005).
Seksek, O., Biwersi, J. & Verkman, A.S. Evidence against defective trans-Golgi acidification in cystic fibrosis. J. Biol. Chem. 271, 15542–15548 (1996).
Haggie, P.M. & Verkman, A.S. Cystic fibrosis transmembrane conductance regulator–independent phagosomal acidification in macrophages. J. Biol. Chem. 282, 31422–31428 (2007).
White, N.M., Corey, D.A. & Kelley, T.J. Mechanistic similarities between cultured cell models of cystic fibrosis and Niemann-Pick type C. Am. J. Respir. Cell Mol. Biol. 31, 538–543 (2004).
Borowitz, D. et al. Gastrointestinal outcomes and confounders in cystic fibrosis. J. Pediatr. Gastroenterol. Nutr. 41, 273–285 (2005).
Cottart, C.H. et al. Impact of nutrition on phenotype in CFTR-deficient mice. Pediatr. Res. 62, 528–532 (2007).
Freedman, S.D. et al. A membrane-lipid imbalance plays a role in the phenotypic expression of cystic fibrosis in Cftr−/− mice. Proc. Natl. Acad. Sci. USA 96, 13995–14000 (1999).
Hariharan, K. & Raina, P.L. Effect of high fat diets with and without cholesterol on erythrocyte and tissue fatty acids in rats. Nahrung 40, 325–330 (1996).
Mosconi, C., Colli, S., Tremoli, E. & Galli, C. Phosphatidylinositol (PI) and PI-associated arachidonate are elevated in platelet total membranes of type IIa hypercholesterolemic subjects. Atherosclerosis 72, 129–134 (1988).
Petrache, I. et al. Ceramide upregulation causes pulmonary cell apoptosis and emphysema-like disease in mice. Nat. Med. 11, 491–498 (2005).
Maiuri, L. et al. DNA fragmentation is a feature of cystic fibrosis epithelial cells: a disease with inappropriate apoptosis? FEBS Lett. 408, 225–231 (1997).
Cannon, C.L., Kowalski, M.P., Stopak, K.S. & Pier, G.B. Pseudomonas aeruginosa–induced apoptosis is defective in respiratory epithelial cells expressing mutant cystic fibrosis transmembrane conductance regulator. Am. J. Respir. Cell Mol. Biol. 29, 188–197 (2003).
Whitchurch, C.B., Tolker–Nielsen, T., Ragas, P.C. & Mattick, J.S. Extracellular DNA required for bacterial biofilm formation. Science 295, 1487 (2002).
Kowalski, M.P. & Pier, G.B. Localization of cystic fibrosis transmembrane conductance regulator to lipid rafts of epithelial cells is required for Pseudomonas aeruginosa–induced cellular activation. J. Immunol. 172, 418–425 (2004).
London, M. & London, E. Ceramide selectively displaces cholesterol from ordered lipid domains (rafts): implications for lipid raft structure and function. J. Biol. Chem. 279, 9997–10004 (2004).
Hogg, J.C. & Senior, R.M. Chronic obstructive pulmonary disease – part 2: pathology and biochemistry of emphysema. Thorax 57, 830–834 (2002).
Forbes, A.R. & Horrigan, R.W. Mucociliary flow in the trachea during anesthesia with enflurane, ether, nitrous oxide, and morphine. Anesthesiology 46, 319–321 (1977).
Acknowledgements
We thank R. Ramphal (University of Florida) for providing P. aeruginosa PAK and PAK mutant strains, G.B. Pier (Channing Laboratory, Brigham and Women's Hospital, Harvard Medical School) for a P. aeruginosa–specific antibody and C. Meisner for statistical evaluations. We thank H. Wegner, M. Niemayer and S. Moyrer for excellent technical assistance. The study was supported by the Deutsche Forschungsgemeinschaft grants Gu 335/10–3/4 and Gu 335/16–1 and the Mukoviszidose e.V. grant F01/04 to E.G.
Author information
Authors and Affiliations
Corresponding author
Supplementary information
Supplementary Text and Figures
Supplementary Notes 1 and 2 and Supplementary Fig. 1 (PDF 327 kb)
Rights and permissions
About this article
Cite this article
Teichgräber, V., Ulrich, M., Endlich, N. et al. Ceramide accumulation mediates inflammation, cell death and infection susceptibility in cystic fibrosis. Nat Med 14, 382–391 (2008). https://doi.org/10.1038/nm1748
Received:
Accepted:
Published:
Issue Date:
DOI: https://doi.org/10.1038/nm1748