Abstract
Tungsten is currently the most promising material for plasma-facing in FRs. The accumulation of radioactive tritium in tungsten is caused by presence of hydrogen traps in this metal. The paper analyzes the literature data on the parameters of hydrogen traps in tungsten. The review results can predict the accumulation of tritium in tungsten cladding of fusion reactors, ensuring their radiation safety.
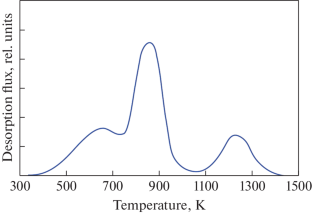
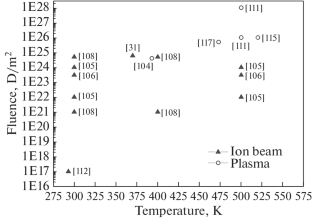
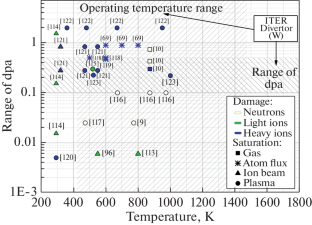

REFERENCES
V. Philipps, P. Mertens, G. F. Matthews, H. Maier, P. Edwards, A. Loving, V. Riccardo, H. Greuner, R. Neu, A. Schmidt, M. Rubel, C. Ruset, E. Villedieu, and JET-EFDA contributors, “Overview of the JET ITER-like Wall Project,” Fusion Eng. Des. 85, 1581–1586 (2010). https://doi.org/10.1016/j.fusengdes.2010.04.048
M. Missirlian, M. Firdaouss, M. Richou, C. Hernandez, L. Gargiulo, J. Bucalossi, C. Brun, Y. Corre, E. Delmas, H. Greuner, B. Guillermin, J. Gunn, J.‑C. C. Hatchressian, R. Jalageas, Q. Li, M. Lipa, M. Lozano, G. Luo, C. Pocheau, H. Roche, E. Tsitrone, N. Vignal, W. Wang, A. Saille, and B. Zago, “Manufacturing, testing and installation of the full tungsten actively cooled ITER-like divertor in the WEST tokamak,” Fusion Eng. Des. 193, 113683 (2022). https://doi.org/10.1016/j.fusengdes.2023.113683
T. Hirai, F. Escourbiac, V. Barabash, A. Durocher, A. Fedosov, L. Ferrand, T. Jokinen, V. Komarov, M. Merola, S. Carpentier-Chouchana, N. Arkhipov, V. Kuznetcov, A. Volodin, S. Suzuki, K. Ezato, Y. Seki, B. Riccardi, M. Bednarek, and P. Gavila, “Status of technology R&D for the ITER tungsten divertor monoblock,” J. Nucl. Mater. 463, 1248–1251 (2015). https://doi.org/10.1016/j.jnucmat.2014.12.027
V. N. Mikhailov, V. A. Evtikhin, I. E. Lyublinskii, A. V. Vertkov, and A. N. Chumanov, Lithium in Thermonuclear Space Energy of the 21st Century (Energoatomizdat, Moscow, 1999).
G. Alefeld and J. Völkl, Hydrogen in Metals I: Basic Properties, Topics in Applied Physics, Vol. 28 (Berlin, 1978).
A. A. Pisarev, Hydrogen Permeability through Metals: A Study Guide (Mosk. Inzh.-Fiz. Inst., Moscow, 2008).
Yu. P. Cherdantsev, I. P. Chernov, and Yu. I. Tyurin, Methods of Investigation of Metal-Hydrogen Systems (Tomsk. Politekh. Univ., Tomsk, 2008).
B. M. Andreev, E. P. Magomedbekov, and M. B. Rozenkevich, “Heterogeneous Tritium Isotope Exchange Reactions,” (1999).
R. A. Causet and T. J. Venhaus, “The use of tungsten in fusion reactors: A review of the hydrogen retention and migration properties,” Phys. Scr. T94, 9 (2001). https://doi.org/10.1238/physica.topical.094a00009
Y. Hatano, M. Shimada, V. Kh. Alimov, J. Shi, M. Hara, T. Nozaki, Y. Oya, M. Kobayashi, K. Okuno, T. Oda, G. Cao, N. Yoshida, N. Futagami, K. Sugiyama, J. Roth, B. Tyburska-Püschel, J. Dorner, I. Takagi, M. Hatakeyama, H. Kurishita, and M. A. Sokolov, “Trapping of hydrogen isotopes in radiation defects formed in tungsten by neutron and ion irradiations,” J. Nucl. Mater. 438, S114–S119 (1010). https://doi.org/10.1016/j.jnucmat.2013.01.018
M. I. Kobayashi, M. Shimada, C. N. Taylor, Yu. Nobuta, Yu. Hatano, and Ya. Oya, “Numerical analysis of deuterium migration behaviors in tungsten damaged by fast neutron by means of gas absorption method,” Fusion Eng. Des. 168, 112635 (2021). https://doi.org/10.1016/j.fusengdes.2021.112635
A. A. van Gorkum and E. V. Kornelsen, “Quantitative thermal desorption spectrometry of ionically implanted inert gases—I. Fundamental aspects,” Vacuum 31, 89–98 (1981). https://doi.org/10.1016/s0042-207x(81)80165-0
E. V. Kornelsen and A. A. van Gorkum, “Quantitative thermal desorption spectrometry of ionically implanted inert gases—II. Technical requirements,” Vacuum 31, 99–111 (1981). https://doi.org/10.1016/s0042-207x(81)80166-2
S. M. Myers, P. M. Richards, W. R. Wampler, and F. Besenbacher, “Ion-beam studies of hydrogen-metal interactions,” J. Nucl. Mater. 165, 9–64 (1989). https://doi.org/10.1016/0022-3115(89)90502-3
O. V. Ogorodnikova, B. Tyburska, V. Kh. Alimov, and K. Ertl, “The influence of radiation damage on the plasma-induced deuterium retention in self-implanted tungsten,” J. Nucl. Mater. 415, S661–S666 (2018). https://doi.org/10.1016/j.jnucmat.2010.12.012
V. S. Efimov, Yu. M. Gasparyan, and A. A. Pisarev, “Investigation of a fine structure of deuterium thermal desorption spectra from tungsten,” J. Surf. Invest. X‑ray, Synchrotron Neutron Tech. 7, 472–478 (2013). https://doi.org/10.1134/s1027451013030051
A. Rusinov, Y. U. Gasparyan, N. Trifonov, A. Pisarev, S. Lindig, and M. Sakamoto, “Investigation of hydrogen-defect interaction in tungsten by the probe fluence method,” J. Nucl. Mater. 415, S645–S648 (2011). https://doi.org/10.1016/j.jnucmat.2010.10.069
I. N. Bekman, Higher Mathematics: Mathematical Apparatus of Diffusion: Textbook for Universities, 2nd ed. (Yurait, Moscow, 2023).
R. Frauenfelder, “Solution and diffusion of hydrogen in tungsten,” J. Vac. Sci. Technol. 6, 388–397 (1919). https://doi.org/10.1116/1.1492699
A. A. Ganeev, A. R. Gubal, S. V. Potapov, N. N. Agafonova, and V. M. Nemets, “Mass spectrometric methods for the direct elemental and isotopic analysis of solid material,” Russ. Chem. Rev. 85, 427–444 (2016). https://doi.org/10.1070/rcr4504
A. Hollingsworth, M. Yu. Lavrentiev, R. Watkins, A. C. Davies, S. Davies, R. Smith, D. R. Mason, A. Baron-Wiechec, Z. Kollo, J. Hess, I. Jepu, J. Likonen, K. Heinola, K. Mizohata, E. Meslin, M. Barthe, A. Widdowson, I. S. Grech, K. Abraham, E. Pender, A. Mcshee, Y. Martynova, M. Freisinger, and A. De Backer, “Comparative study of deuterium retention in irradiated Eurofer and Fe–Cr from a new ion implantation materials facility,” Nucl. Fusion 60, 016024 (2019). https://doi.org/10.1088/1741-4326/ab546e
A. A. Haasz, M. Poon, R. G. Macaulay-Newcombe, and J. W. Davis, “Deuterium retention in single crystal tungsten,” J. Nucl. Mater. 290-293, 85–88 (2001). https://doi.org/10.1016/s0022-3115(00)00615-2
J. P. Coad, J. Likonen, M. Rubel, E. Vainonen-Ahlgren, D. E. Hole, T. Sajavaara, T. Renvall, G. F. Matthews, and J. E. Contributors, “Overview of material re-deposition and fuel retention studies at JET with the Gas Box divertor,” Nucl. Fusion 46, 350–366 (2006). https://doi.org/10.1088/0029-5515/46/2/018
J. Karhunen, A. Hakola, J. Likonen, A. Lissovski, M. Laan, and P. Paris, “Applicability of LIBS for in situ monitoring of deposition and retention on the ITER-like wall of JET—Comparison to SIMS,” J. Nucl. Mater. 463, 931–935 (2015). https://doi.org/10.1016/j.jnucmat.2014.10.028
M. Mayer, V. Rohde, J. Likonen, E. Vainonen-Ahlgren, K. Krieger, X. Gong, J. Chen et al. (ASDEX Upgrade Team. Carbon), “Carbon erosion and deposition on the ASDEX Upgrade divertor tiles,” J. Nucl. Mater. 337–339, 119–123 (2005). https://doi.org/10.1016/j.jnucmat.2004.10.046
F. Pavlyak, “Investigation of hydrogen sensitivity of SIMS method,” Surf. Interface Anal. 19, 232–236 (1992). https://doi.org/10.1002/sia.740190145
M. Wilde and K. Fukutani, “Hydrogen detection near surfaces and shallow interfaces with resonant nuclear reaction analysis,” Surf. Sci. Rep. 69, 196–295 (2014). https://doi.org/10.1016/j.surfrep.2014.08.002
M. Mayer, SIMNRA User’s Guide (1997).
V. Kh. Alimov, J. Roth, and M. Mayer, “Depth distribution of deuterium in single- and polycrystalline tungsten up to depths of several micrometers,” J. Nucl. Mater. 337–339, 619–623 (2005). https://doi.org/10.1016/j.jnucmat.2004.10.082
R. A. Langley, S. T. Picraux, and F. L. Vook, “Depth distribution profiling of deuterium and 3He,” J. Nucl. Mater. 53, 257–261 (1974). https://doi.org/10.1016/0022-3115(74)90253-0
V. Kh. Alimov, M. Mayer, and J. Roth, “Differential cross-section of the D(3He,p)4He nuclear reaction and depth profiling of deuterium up to large depths,” Nucl. Instrum. Methods Phys. Res., Sect. B 234, 169–175 (2005). https://doi.org/10.1016/j.nimb.2005.01.009
A. Manhard, “Deuterium inventory in tungsten after plasma exposure: a microstructural survery,” PhD Thesis (Univ. Augsburg, Augsburg, Germany, 2012).
G. M. Wright, D. G. Whyte, and B. Lipschultz, “Measurement of hydrogenic retention and release in molybdenum with the DIONISOS experiment,” J. Nucl. Mater. 390–391, 544–549 (2009). https://doi.org/10.1016/j.jnucmat.2009.01.092
V. Kh. Alimov, N. P. Bobyr, A. V. Spitsyn, and D. I. Cherkez, “Deuterium retention in radiation-damaged tungsten,” Vopr. At. Nauki Tekh. Ser.: Termoyadernyi Sintez 40 (4), 25–39 (2017). https://doi.org/10.21517/0202-3822-2017-40-4-25-39
P. E. Lhuillier, T. Belhabib, P. Desgardin, B. Courtois, T. Sauvage, M. F. Barthe, A. L. Thomann, P. Brault, and Y. Tessier, “Helium retention and early stages of helium-vacancy complexes formation in low energy helium-implanted tungsten,” J. Nucl. Mater. 433, 305–313 (2013). https://doi.org/10.1016/j.jnucmat.2012.09.001
I. Takagi, S. Nomura, T. Minamimoto, M. Akiyoshi, T. Kobayashi, and T. Sasaki, “Hydrogen–deuterium exchange on plasma-exposed W and SS surface,” J. Nucl. Mater. 463, 1125–1128 (2015). https://doi.org/10.1016/j.jnucmat.2014.11.037
V. Kh. Alimov, B. Tyburska-Püschel, M. H. J. ‘t Hoen, J. Roth, Y. Hatano, K. Isobe, M. Matsuyama, and T. Yamanishi, “Hydrogen isotope exchange in tungsten irradiated sequentially with low-energy deuterium and protium ions,” Phys. Scr. T145, 014037 (2011). https://doi.org/10.1088/0031-8949/2011/t145/014037
O. V. Ogorodnikova, L. Yu. Dubov, S. V. Stepanov, D. Terentyev, Yu. V. Funtikov, Yu. V. Shtotsky, V. S. Stolbunov, V. Efimov, and K. Gutorov, “Annealing of radiation-induced defects in tungsten: Positron annihilation spectroscopy study,” J. Nucl. Mater. 517, 148–151 (2019). https://doi.org/10.1016/j.jnucmat.2019.02.010
F. A. Selim, “Positron annihilation spectroscopy of defects in nuclear and irradiated materials–A review,” Mater. Charact. 174, 110952 (2021). https://doi.org/10.1016/j.matchar.2021.110952
A. Dupasquier and M. J. Manninen, Positrons in Solids, Topics in Current Physics, Vol. 12 (Springer, Berlin, 1979). https://doi.org/10.1007/978-3-642-81316-0
M. Eldrup and B. N. Singh, “Studies of defects and defect agglomerates by positron annihilation spectroscopy,” J. Nucl. Mater. 251, 132–138 (1997). https://doi.org/10.1016/s0022-3115(97)00221-3
Principles and Applications of Positron and Positronium Chemistry, Ed. by Y. C. Jean, P. E. Mallon, and D. M. Schrader (World Scientific, Singapore, 2003). https://doi.org/10.1142/9789812775610
W. M. Shu, A. Kawasuso, Y. Miwa, E. Wakai, G. Luo, and T. Yamanishi, “Microstructure dependence of deuterium retention and blistering in the near-surface region of tungsten exposed to high flux deuterium plasmas of 38 eV at 315 K,” Phys. Scr. T128, 96–99 (2007). https://doi.org/10.1088/0031-8949/2007/t128/019
H. Eleveld and A. Van Veen, “Void growth and thermal desorption of deuterium from voids in tungsten,” J. Nucl. Mater. 212–215, 1421–1425 (1994). https://doi.org/10.1016/0022-3115(94)91062-6
A. Debelle, M. F. Barthe, and T. Sauvage, “First temperature stage evolution of irradiation-induced defects in tungsten studied by positron annihilation spectroscopy,” J. Nucl. Mater. 376, 216–221 (2008). https://doi.org/10.1016/j.jnucmat.2008.03.002
S. Zhu, Yo. Xu, Z. Wang, Yo. Zheng, D. Zhou, E. Du, D. Yuan, M. Fukuda, M. Mihara, K. Matsuta, and T. Minamisono, “Positron annihilation lifetime spectroscopy on heavy ion irradiated stainless steels and tungsten,” J. Nucl. Mater. 343, 330–332 (2005). https://doi.org/10.1016/j.jnucmat.2004.11.024
F. Liu, Yu. Xu, H. Zhou, X. Li, Yi. Song, C. Zhang, Q. Li, C. He, and G.-N. Luo, “Defect production and deuterium retention in quasi-homogeneously damaged tungsten,” Nucl. Instrum. Methods Phys. Res., Sect. B 351, 23–26 (2015). https://doi.org/10.1016/j.nimb.2015.03.080
M. Zibrov, W. Egger, J. Heikinheimo, M. Mayer, and F. Tuomisto, “Vacancy cluster growth and thermal recovery in hydrogen-irradiated tungsten,” J. Nucl. Mater. 531, 152017 (2020). https://doi.org/10.1016/j.jnucmat.2020.152017
J. I. Goldstein, D. E. Newbury, P. Echlin, D. C. Joy, A. D. Romig, C. E. Lyman, C. Fiori, and E. Lifshin, Scanning Electron Microscopy and X-Ray Microanalysis: A Text for Biologists, Materials Scientists, and Geologists (Springer, New York, 1981). https://doi.org/10.1007/978-1-4613-0491-3
S. R. Falsafi, H. Rostamabadi, E. Assadpour, and S. M. Jafari, “Morphology and microstructural analysis of bioactive-loaded micro/nanocarriers via microscopy techniques; CLSM/SEM/TEM/AFM,” Adv. Colloid Interface Sci. 280, 102166 (2020). https://doi.org/10.1016/j.cis.2020.102166
A. Manhard, G. Matern, and M. Balden, “A step-by-step analysis of the polishing process for tungsten specimens,” Pract. Metallogr. 50, 5–16 (2013). https://doi.org/10.3139/147.110215
A. V. Golubeva, V. A. Kurnaev, M. Mayer, and J. Roth, “Deuterium capture in plasma-sprayed tungsten,”, No. 2, 18–25 (2007).
Y. U. Gasparyan, M. Rasinski, M. Mayer, A. Pisarev, and J. Roth, “Deuterium ion-driven permeation and bulk retention in tungsten,” J. Nucl. Mater. 417, 540–544 (2011). https://doi.org/10.1016/j.jnucmat.2010.12.119
Yu. M. Gasparyan, “Capture and outgassing of deuterium during ionic introduction into tungsten,” Candidate’s Dissertation in Physics and Mathematics (National Research Nuclear Univ. MEPhI, Moscow, 2009).
Umanskii Ya S, Yu. A. Skakov, A. N. Ivanov, and L. N. Rastorguev, Crystallography, X-Ray and Electron Microscopy (Metallurgiya, Moscow, 1982).
L. Reimer, Transmission Electron Microscopy: Physics of Image Formation and Microanalysis, Springer Series in Optical Sciences, Vol. 36 (Springer, Berlin, 2013). https://doi.org/10.1007/978-3-662-14824-2
B. I. Khripunov, V. S. Koidan, A. I. Ryazanov, V. M. Gureev, S. N. Kornienko, S. T. Latushkin, A. M. Muksunov, E. V. Semenov, V. G. Stolyarova, and V. N. Unezhev, “Radiation-damaged tungsten: Production and study in a steady-state plasma flux,” Phys. At. Nucl. 81, 1015–1023 (2017). https://doi.org/10.1134/s1063778818070049
M. Zibrov, M. Balden, M. Dickmann, A. Dubinko, W. Egger, M. Mayer, D. Terentyev, and M. Wirtz, “Deuterium trapping by deformation-induced defects in tungsten,” Nucl. Fusion 59, 106056 (2019). https://doi.org/10.1088/1741-4326/ab3c7e
W. Chrominski, L. Ciupinski, P. Bazarnik, S. Markelj, and T. Schwarz-Selinger, “TEM investigation of the influence of dose rate on radiation damage and deuterium retention in tungsten,” Mater. Charact. 154, 1–6 (2019). https://doi.org/10.1016/j.matchar.2019.05.028
M. Pečovnik, “The influence of hydrogen isotopes on the behaviour of crystal lattice defects in tungsten,” PhD Dissertation (Univerza v Ljubljani, Ljubljana, 2021).
P. Wang, Q. Cao, J. Hou, X. Kong, L. Chen, and Z. M. Xie, “Implantation and desorption of H isotopes in W revisited by object kinetic Monte Carlo simulation,” J. Nucl. Mater. 561, 153576 (2022). https://doi.org/10.1016/j.jnucmat.2022.153576
C. S. Becquart, C. Domain, U. Sarkar, A. Debacker, and M. Hou, “Microstructural evolution of irradiated tungsten: Ab initio parameterisation of an OKMC model,” J. Nucl. Mater. 403, 75–88 (2010). https://doi.org/10.1016/j.jnucmat.2010.06.003
F. Jiménez and C. J. Ortiz, “A GPU-based parallel object kinetic Monte Carlo algorithm for the evolution of defects in irradiated materials,” Comput. Mater. Sci. 113, 178–186 (2016). https://doi.org/10.1016/j.commatsci.2015.11.011
A. McNabb and P. K. Foster, “A new analysis of the diffusion of hydrogen in iron and ferritic steels,” Trans. Met. Soc. AIME 227, 618–627 (1963).
S. M. Myers, W. R. Wampler, and F. Besenbacher, “Trapping and surface recombination of ion-implanted deuterium in stainless steel,” J. Appl. Phys. 56, 1561–1571 (1984). https://doi.org/10.1063/1.334165
G. R. Longhurst, TMAP7 User Manual (Idaho Natl. Lab. (INL), 2008). https://doi.org/10.2172/952013
B. J. Merrill, M. Shimada, and P. W. Humrickhouse, “Simulating tritium retention in tungsten with a multiple trap model in the TMAP code,” J. Plasma Fusion Res. SERIES 10, 71–75 (2013).
M. J. Simmonds, “Investigating fusion relevant plasma material interactions: Analyzing hydrogenic isotope retention in heavy-ion damaged tungsten,” PhD Dissertation (Univ. of California, San Diego, Calif., 2018).
Yu. M. Gasparyan, O. V. Ogorodnikova, V. S. Efimov, A. Mednikov, E. D. Marenkov, A. A. Pisarev, S. Markelj, and I. Čadež, “Thermal desorption from self-damaged tungsten exposed to deuterium atoms,” J. Nucl. Mater. 463, 1013–1016 (2015). https://doi.org/10.1016/j.jnucmat.2014.11.022
J. Guterl, R. D. Smirnov, S. I. Krasheninnikov, M. Zibrov, and A. A. Pisarev, “Theoretical analysis of deuterium retention in tungsten plasma-facing components induced by various traps via thermal desorption spectroscopy,” Nucl. Fusion 55, 093017 (2015). https://doi.org/10.1088/0029-5515/55/9/093017
Ya. Oya, X. Li, M. Sato, K. Yuyama, L. Zhang, S. Kondo, T. Hinoki, Yu. Hatano, H. Watanabe, N. Yoshida, and T. Chikada, “Thermal desorption behavior of deuterium for 6 MeV Fe ion irradiated W with various damage concentrations,” J. Nucl. Mater. 461, 336–340 (2015). https://doi.org/10.1016/j.jnucmat.2015.03.032
M. A. Pick and K. Sonnenberg, “A model for atomic hydrogen-metal interactions—Application to recycling, recombination and permeation,” J. Nucl. Mater. 131, 208–220 (1985). https://doi.org/10.1016/0022-3115(85)90459-3
O. V. Ogorodnikova, J. Roth, and M. Mayer, “Deuterium retention in tungsten in dependence of the surface conditions,” J. Nucl. Mater. 313–316, 469–477 (2003). https://doi.org/10.1016/s0022-3115(02)01375-2
A. Hu and A. Hassanein, “Modeling hydrogen isotope behavior in fusion plasma-facing components,” J. Nucl. Mater. 446, 56–62 (2014). https://doi.org/10.1016/j.jnucmat.2013.11.033
A. C. Hindmarsh, P. N. Brown, K. E. Grant, S. L. Lee, R. Serban, D. E. Shumaker, and C. S. Woodward, “SUNDIALS: Suite of nonlinear and differential/algebraic equation solvers,” ACM Trans. Math. Software 31, 363–396 (2005). https://doi.org/10.1145/1089014.1089020
T. Ahlgren, K. Heinola, K. Vörtler, and J. Keinonen, “Simulation of irradiation induced deuterium trapping in tungsten,” J. Nucl. Mater. 427, 152–161 (2012). https://doi.org/10.1016/j.jnucmat.2012.04.031
P. Grigorev, D. Matveev, A. Bakaeva, D. Terentyev, E. E. Zhurkin, G. Van Oost, and J.-M. Noterdaeme, “Modelling deuterium release from tungsten after high flux high temperature deuterium plasma exposure,” J. Nucl. Mater. 481, 181–189 (2016). https://doi.org/10.1016/j.jnucmat.2016.09.019
C. Sang, X. Bonnin, M. Warrier, A. Rai, R. Schneider, J. Sun, and D. Wang, “Modelling of hydrogen isotope inventory in mixed materials including porous deposited layers in fusion devices,” Nucl. Fusion 52, 043003 (2012). https://doi.org/10.1088/0029-5515/52/4/043003
Z. Wang, C. Sang, and D. Wang, “Modelling of deuterium retention and outgassing in self-damaged tungsten under low-energy atomic D flux irradiation: The effects of surface processes,” J. Nucl. Mater. 540, 152390 (2020). https://doi.org/10.1016/j.jnucmat.2020.152390
M. Oberkofler, M. Reinelt, and C. H. Linsmeier, “Retention and release mechanisms of deuterium implanted into beryllium,” Nucl. Instrum. Methods Phys. Res., Sect. B 269, 1266–1270 (2011). https://doi.org/10.1016/j.nimb.2010.11.058
D. Matveev, M. Wensing, S. Möller, A. Kreter, S. Brezinsek, and C. Linsmeier, “Hydrogen outgassing following plasma exposure,” (EUROFUSION WPPFC-CP(16), 2016), p. 14877.
L. Buzi, G. De Temmerman, B. Unterberg, M. Reinhart, T. Dittmar, D. Matveev, C. H. Linsmeier, U. Breuer, A. Kreter, and G. Van Oost, “Influence of tungsten microstructure and ion flux on deuterium plasma-induced surface modifications and deuterium retention,” J. Nucl. Mater. 463, 320–324 (2015). https://doi.org/10.1016/j.jnucmat.2014.12.006
R. Piechoczek, M. Reinelt, M. Oberkofler, A. Allouche, and C. H. Linsmeier, “Deuterium trapping and release in Be(0001), Be(11–20) and polycrystalline beryllium,” J. Nucl. Mater. 438, S1072–S1075 (2013). https://doi.org/10.1016/j.jnucmat.2013.01.235
E. A. Hodille, X. Bonnin, R. Bisson, T. Angot, C. S. Becquart, J. M. Layet, and C. Grisolia, “Macroscopic rate equation modeling of trapping/detrapping of hydrogen isotopes in tungsten materials,” J. Nucl. Mater. 467, 424–431 (2015). https://doi.org/10.1016/j.jnucmat.2015.06.041
E. A. Hodille, Y. Ferro, N. Fernandez, C. S. Becquart, T. Angot, J. M. Layet, R. Bisson, and C. Grisolia, “Study of hydrogen isotopes behavior in tungsten by a multi trapping macroscopic rate equation model,” Phys. Scr. T167, 014011 (2016). https://doi.org/10.1088/0031-8949/2016/t167/014011
E. A. Hodille, S. Markelj, M. Pecovnik, M. Ajmalghan, Z. A. Piazza, Y. Ferro, T. Schwarz-Selinger, and C. Grisolia, “Kinetic model for hydrogen absorption in tungsten with coverage dependent surface mechanisms,” Nucl. Fusion 60, 106011 (2020). https://doi.org/10.1088/1741-4326/aba454
M. Pečovnik, T. Schwarz-Selinger, and S. Markelj, “Experiments and modelling of multiple sequential MeV ion irradiations and deuterium exposures in tungsten,” J. Nucl. Mater. 550, 152947 (2021). https://doi.org/10.1016/j.jnucmat.2021.152947
R. Delaporte-Mathurin, E. A. Hodille, J. Mougenot, Ya. Charles, and C. Grisolia, “Finite element analysis of hydrogen retention in ITER plasma facing components using FESTIM,” Nucl. Mater. Energy 21, 100709 (2019). https://doi.org/10.1016/j.nme.2019.100709
S. Benannoune, Y. Charles, J. Mougenot, M. Gaspérini, and G. De Temmerman, “Numerical simulation by finite element modelling of diffusion and transient hydrogen trapping processes in plasma facing components,” Nucl. Mater. Energy 19, 42–46 (2019). https://doi.org/10.1016/j.nme.2019.01.023
ABAQUS User Subroutines Reference Manual (Simulia, Dassault Système, 2011).
K. Schmid, V. Rieger, and A. Manhard, “Comparison of hydrogen retention in W and W/Ta alloys,” J. Nucl. Mater. 426, 247–253 (2012). https://doi.org/10.1016/j.jnucmat.2012.04.003
K. Schmid, “Diffusion-trapping modelling of hydrogen recycling in tungsten under ELM-like heat loads,” Phys. Scr. T167, 014025 (2016). https://doi.org/10.1088/0031-8949/t167/1/014025
K. Schmid, U. Von Toussaint, and T. Schwarz-Selinger, “Transport of hydrogen in metals with occupancy dependent trap energies,” J. Appl. Phys. 116 (2014). https://doi.org/10.1063/1.4896580
K. Schmid and M. Zibrov, “On the use of recombination rate coefficients in hydrogen transport calculations,” Nucl. Fusion 61, 086008 (2021). https://doi.org/10.1088/1741-4326/ac07b2
H. E. Kissinger, “Variation of peak temperature with heating rate in differential thermal analysis,” J. Res. Natl. Bureau Standards 57, 217–221 (1956). https://doi.org/10.6028/jres.057.026
M. Zibrov, S. Ryabtsev, Y. U. Gasparyan, and A. Pisarev, “Experimental determination of the deuterium binding energy with vacancies in tungsten,” J. Nucl. Mater. 477, 292–297 (2016). https://doi.org/10.1016/j.jnucmat.2016.04.052
F. Wei, M. Enomoto, and K. Tsuzaki, “Applicability of the Kissinger’s formula and comparison with the McNabb–Foster model in simulation of thermal desorption spectrum,” Comput. Mater. Sci. 51, 322–330 (2012). https://doi.org/10.1016/j.commatsci.2011.07.009
L. Cheng, M. Enomoto, and F.-G. Wei, “Further assessment of the Kissinger formula in simulation of thermal desorption spectrum of hydrogen,” ISIJ Int. 53, 250–256 (2013). https://doi.org/10.2355/isijinternational.53.250
M. S. Zibrov, A. S. Shubina, Yu. M. Gasparyan, and A. A. Pisarev, “On the possibility of determination of the hydrogen binding energies with defects from thermal desorption measurements with different heating rates,” Vopr. At. Nauki Tekh. Ser.: Termoyadernyi Sintez 38 (1), 32–41 (2015). https://doi.org/10.21517/0202-3822-2015-38-1-32-41
R. A. Oriani, “The diffusion and trapping of hydrogen in steel,” Acta Metall. 18, 147–157 (1970). https://doi.org/10.1016/0001-6160(70)90078-7
T. A. Shishkova, A. V. Golubeva, and M. B. Rozenkevich, “Isotope effect in the interaction between hydrogen and fusion reactor materials,” Russ. J. Phys. Chem. A 97, 2079–2098 (2023). https://doi.org/10.1134/s0036024423100205
F. Liu, H. Zhou, X.-Ch. Li, Yu. Xu, Z. An, H. Mao, W. Xing, Q. Hou, and G.-N. Luo, “Deuterium gas-driven permeation and subsequent retention in rolled tungsten foils,” J. Nucl. Mater. 455, 248–252 (2014). https://doi.org/10.1016/j.jnucmat.2014.06.005
X. Ye, G. Li, C. Liang, B. Ma, X. Xiang, Z. Zheng, M. Ni, and C. Chen, “Determination of the detrapping energy of tritium in tungsten,” J. Nucl. Mater. 544, 152662 (2021). https://doi.org/10.1016/j.jnucmat.2020.152662
X. Ye, W. Wang, Q. Pan, C. Jiang, Yi. Wang, X. Chen, J. Wu, and C. Chen, “Comparison of deuterium retention in tungsten exposed to deuterium plasma and gas,” Nucl. Mater. Energy 24, 100775 (2020). https://doi.org/10.1016/j.nme.2020.100775
M. Poon, A. A. Haasz, and J. W. Davis, “Modelling deuterium release during thermal desorption of D+-irradiated tungsten,” J. Nucl. Mater. 374, 390–402 (2008). https://doi.org/10.1016/j.jnucmat.2007.09.028
J. P. Roszell, J. W. Davis, and A. A. Haasz, “Temperature dependence of deuterium retention mechanisms in tungsten,” J. Nucl. Mater. 429, 48–54 (2012). https://doi.org/10.1016/j.jnucmat.2012.05.018
R. A. Anderl, D. F. Holland, G. R. Longhurst, R. J. Pawelko, C. L. Trybus, and C. H. Sellers, “Deuterium transport and trapping in polycrystalline tungsten,” Fusion Technol. 21, 745–752 (1992). https://doi.org/10.13182/fst92-a29837
O. V. Ogorodnikova, J. Roth, and M. Mayer, “Ion-driven deuterium retention in tungsten,” J. Appl. Phys. 103, 34902 (2008). https://doi.org/10.1063/1.2828139
H. Nakamura, T. Hayashi, Ya. Iwai, and M. Nishi, “The effect of annealing on the transient deuterium permeation characteristics of tungsten,” Fusion Technol. 39, 894–898 (2001). https://doi.org/10.13182/fst01-a11963353
O. V. Ogorodnikova, K. Sugiyama, T. Schwarz-Selinger, T. Dürbeck, and M. Balden, “Ion-induced deuterium retention in tungsten coatings on carbon substrate,” J. Nucl. Mater. 419, 194–200 (2011). https://doi.org/10.1016/j.jnucmat.2011.07.023
M. Liu, W. Guo, L. Cheng, J. Wang, S. Wang, H. Yin, T. Wang, Yu. Huang, Yu. Yuan, T. Schwarz-Selinger, G. D. Temmerman, X.-Zh. Cao, G.-N. Luo, and G.‑H. Lu, “Blister-dominated retention mechanism in tungsten exposed to high-fluence deuterium plasma,” Nucl. Fusion 60, 126034 (2020). https://doi.org/10.1088/1741-4326/abb600
H. Eleveld and A. Van Veen, “Deuterium interaction with impurities in tungsten studied with TDS,” J. Nucl. Mater. 191–194, 433–438 (1992). https://doi.org/10.1016/s0022-3115(09)80082-2
S. Ryabtsev, Y. U. Gasparyan, M. Zibrov, A. Shubina, and A. Pisarev, “Deuterium thermal desorption from vacancy clusters in tungsten,” Nucl. Instrum. Methods Phys. Res., Sect. B 382, 101–104 (2016). https://doi.org/10.1016/j.nimb.2016.04.038
Z. Harutyunyan, Yu. Gasparyan, S. Ryabtsev, V. Efimov, O. Ogorodnikova, A. Pisarev, and S. Kanashenko, “Deuterium trapping in the subsurface layer of tungsten pre-irradiated with helium ions,” J. Nucl. Mater. 548, 152848 (2021). https://doi.org/10.1016/j.jnucmat.2021.152848
Sh.-Ya. Qin, S. Jin, D.-R. Zou, L. Cheng, X.-L. Shu, Q. Hou, and G.-H. Lu, “The effect of inert gas pre-irradiation on the retention of deuterium in tungsten: A TMAP investigation combined with first-principles method,” Fusion Eng. Des. 121, 342–347 (2017). https://doi.org/10.1016/j.fusengdes.2017.04.120
Y. Oya, F. Sun, Y. Yamauchi, Y. Nobuta, M. Shimada, C. N. Taylor, W. R. Wampler, M. Nakata, L. M. Garrison, and Y. Hatano, “D retention and depth profile behavior for single crystal tungsten with high temperature neutron irradiation,” J. Nucl. Mater. 539, 152323 (2020). https://doi.org/10.1016/j.jnucmat.2020.152323
M. Shimada, G. Cao, Y. Hatano, T. Oda, Y. Oya, M. Hara, and P. Calderoni, “The deuterium depth profile in neutron-irradiated tungsten exposed to plasma,” Phys. Scr. T145, 014051 (2011). https://doi.org/10.1088/0031-8949/2011/t145/014051
E. A. Hodille, A. Založnik, S. Markelj, T. Schwarz-Selinger, C. S. Becquart, R. Bisson, and C. Grisolia, “Simulations of atomic deuterium exposure in self-damaged tungsten,” Nucl. Fusion 57, 056002 (2017). https://doi.org/10.1088/1741-4326/aa5aa5
E. A. Hodille, S. Markelj, T. Schwarz-Selinger, A. Založnik, M. Pečovnik, M. Kelemen, and C. Grisolia, “Stabilization of defects by the presence of hydrogen in tungsten: Simultaneous W-ion damaging and D‑atom exposure,” Nucl. Fusion 59, 016011 (2018). https://doi.org/10.1088/1741-4326/aaec97
J. R. Fransens, M. S. Abd El Keriem, and F. Pleiter, “Hydrogen-vacancy interaction in tungsten,” J. Phys.: Condens. Matter 3, 9871–9886 (1991). https://doi.org/10.1088/0953-8984/3/49/004
O. V. Ogorodnikova, B. Tyburska, V. Kh. Alimov, and K. Ertl, “The influence of radiation damage on the plasma-induced deuterium retention in self-implanted tungsten,” J. Nucl. Mater. 415, S661–S666 (2011). https://doi.org/10.1016/j.jnucmat.2010.12.012
G. M. Wright, M. Mayer, K. Ertl, G. de Saint-Aubin, and J. Rapp, “TMAP7 simulations of deuterium trapping in pre-irradiated tungsten exposed to high-flux plasma,” J. Nucl. Mater. 415, S636–S640 (2011). https://doi.org/10.1016/j.jnucmat.2011.01.011
M. H. J. Hoen, M. Mayer, A. W. Kleyn, H. Schut, and P. A. Zeijlmans van Emmichoven, “Reduced deuterium retention in self-damaged tungsten exposed to high-flux plasmas at high surface temperatures,” Nucl. Fusion 53, 43003 (2013).
A. D. Quastel, J. W. Davis, A. A. Haasz, and R. G. Macaulay-Newcombe, “Effect of post-D+-irradiation time delay and pre-TDS heating on D retention in single crystal tungsten,” J. Nucl. Mater. 359, 8–16 (2006). https://doi.org/10.1016/j.jnucmat.2006.07.012
H. Nakamura, T. Hayashi, M. Nishi, M. Arita, and K. Okuno, “Implantation driven permeation behavior of deuterium through pure tungsten,” Fusion Eng. Des. 55, 513–520 (2001). https://doi.org/10.1016/s0920-3796(01)00380-5
M. Kobayashi, M. Shimada, C. N. Taylor, D. Buchenauer, R. Kolasinski, T. Koyanagi, Yu. Nobuta, Yu. Hatano, and Ya. Oya, “Influence of dynamic annealing of irradiation defects on the deuterium retention behaviors in tungsten irradiated with neutron,” Fusion Eng. Des. 146, 1624–1627 (2019). https://doi.org/10.1016/j.fusengdes.2019.03.003
A. Založnik, S. Markelj, T. Schwarz-Selinger, Ł. Ciu-piński, J. Grzonka, P. Vavpetič, and P. Pelicon, “The influence of the annealing temperature on deuterium retention in self-damaged tungsten,” Phys. Scr. T167, 014031 (2016). https://doi.org/10.1088/0031-8949/t167/1/014031
S. Markelj, A. Založnik, T. Schwarz-Selinger, O. V. Ogorodnikova, P. Vavpetič, P. Pelicon, and I. Čadež, “In situ NRA study of hydrogen isotope exchange in self-ion damaged tungsten exposed to neutral atoms,” J. Nucl. Mater. 469, 133–144 (2016). https://doi.org/10.1016/j.jnucmat.2015.11.039
S. Krat, Y. U. Gasparyan, Y. A. Vasina, A. Davletiyarova, and A. Pisarev, “Tungsten-deuterium co-deposition: Experiment and analytical description,” Vacuum 149, 23–28 (2018). https://doi.org/10.1016/j.vacuum.2017.12.004
K. Kremer, M. Brucker, W. Jacob, and T. Schwarz-Selinger, “Influence of thin surface oxide films on hydrogen isotope release from ion-irradiated tungsten,” Nucl. Mater. Energy 30, 101137 (2022). https://doi.org/10.1016/j.nme.2022.101137
K. A. Moshkunov, K. Schmid, M. Mayer, V. A. Kurnaev, and Yu. M. Gasparyan, “Air exposure and sample storage time influence on hydrogen release from tungsten,” J. Nucl. Mater. 404, 174–177 (2010). https://doi.org/10.1016/j.jnucmat.2010.07.011
Yu. V. Zaika, E. K. Kostikova, and Yu. S. Nechaev, “Peaks of hydrogen thermal desorption: Simulation and interpretation,” Tech. Phys. 66, 210–220 (2021). https://doi.org/10.1134/S1063784221020250
J. Roth, E. Tsitrone, T. Loarer, V. Philipps, S. Brezinsek, A. Loarte, G. F. Counsell, R. P. Doerner, K. Schmid, O. V. Ogorodnikova, and R. A. Causey, “Tritium inventory in ITER plasma-facing materials and tritium removal procedures,” Plasma Phys. Controlled Fusion 50, 103001 (2008). https://doi.org/10.1088/0741-3335/50/10/103001
J. H. You, E. Visca, T. Barrett, B. Böswirth, F. Crescenzi, F. Domptail, M. Fursdon, F. Gallay, B.‑E. Ghidersa, H. Greuner, M. Li, A. V. Müller, J. Reiser, M. Richou, S. Roccella, and C. H. Vorpahl, “European divertor target concepts for DEMO: Design rationales and high heat flux performance,” Nucl. Mater. Energy 16, 1–11 (2018). https://doi.org/10.1016/j.nme.2018.05.012
R. Villari, V. Barabash, F. Escourbiac, L. Ferrand, T. Hirai, V. Komarov, M. Loughlin, M. Merola, F. Moro, L. Petrizzi, S. Podda, E. Polunovsky, and G. Brolatti, “Nuclear analysis of the ITER full-tungsten divertor,” Fusion Eng. Des. 88, 2006–2010 (2006). https://doi.org/10.1016/j.fusengdes.2013.02.156
J. Roth, E. Tsitrone, A. Loarte, T. H. Loarer, G. Counsell, R. Neu, V. Philipps, S. Brezinsek, M. Lehnen, P. Coad, C. H. Grisolia, K. Schmid, K. Krieger, A. Kallenbach, B. Lipschultz, R. Doerner, R. Causey, V. Alimov, W. Shu, O. Ogorodnikova, A. Kirschner, G. Federici, A. Kukushkin, and EFDA PWI Task Force, ITER PWI Team, Fusion for Energy, ITPA SOL/DIV, “Recent analysis of key plasma wall interactions issues for ITER,” J. Nucl. Mater. 390-391, 1–9 (2009). https://doi.org/10.1016/j.jnucmat.2009.01.037
O. V. Ogorodnikova and K. Sugiyama, “Effect of radiation-induced damage on deuterium retention in tungsten, tungsten coatings and Eurofer,” J. Nucl. Mater. 442, 518–527 (2013). https://doi.org/10.1016/j.jnucmat.2013.07.024
V. P. Budaev, “Results of high heat flux tests of tungsten divertor targets under plasma heat loads expected in ITER and tokamaks (review),” Phys. At. Nucl. 79, 1137–1162 (2016). https://doi.org/10.1134/S106377881607005X
G.-H. Lu, H.-B. Zhou, and C. S. Becquart, “A review of modelling and simulation of hydrogen behaviour in tungsten at different scales,” Nucl. Fusion 54, 086001 (2014). https://doi.org/10.1088/0029-5515/54/8/086001
L. Gao, M. Wilde, A. Manhard, U. Von Toussaint, and W. Jacob, “Hydrogen atom-ion synergy in surface lattice modification at sub-threshold energy,” Acta Mater. 201, 55–62 (2020). https://doi.org/10.1016/j.actamat.2020.09.065
L. Gao, W. Jacob, U. Von Toussaint, A. Manhard, M. Balden, K. Schmid, and T. Schwarz-Selinger, “Deuterium supersaturation in low-energy plasma-loaded tungsten surfaces,” Nucl. Fusion 57, 016026 (2016). https://doi.org/10.1088/0029-5515/57/1/016026
A. Založnik, S. Markelj, T. Schwarz-Selinger, and K. Schmid, “Deuterium atom loading of self-damaged tungsten at different sample temperatures,” J. Nucl. Mater. 496, 1–8 (2017). https://doi.org/10.1016/j.jnucmat.2017.09.003
V. K. Alimov and J. Roth, “Hydrogen isotope retention in plasma-facing materials: Review of recent experimental results,” Phys. Scr. T128, 6–13 (2007). https://doi.org/10.1088/0031-8949/2007/t128/002
V. Kh. Alimov, Y. Hatano, K. Sugiyama, J. Roth, B. Tyburska-Püschel, J. Dorner, J. Shi, M. Matsuyama, K. Isobe, and T. Yamanishi, “The effect of displacement damage on deuterium retention in tungsten exposed to D neutrals and D2 gas,” J. Nucl. Mater. 438, S959–S962 (2013). https://doi.org/10.1016/j.jnucmat.2013.01.208
V. Kh. Alimov, Y. Hatano, B. Tyburska-Püschel, K. Sugiyama, I. Takagi, Y. Furuta, J. Dorner, M. Fußeder, K. Isobe, T. Yamanishi, and M. Matsuyama, “Deuterium retention in tungsten damaged with W ions to various damage levels,” J. Nucl. Mater. 441, 280–285 (2009). https://doi.org/10.1016/j.jnucmat.2013.06.005
Z. R. Arutyunyan, “Effect of helium on the capture of deuterium ions in tungsten and tungsten alloys W Cr-Y,” Candidate’s Dissertation in Physics and Mathematics (National Research Nuclear Univ. MEPhI, Moscow, 2022).
T. Muroga, Y. Hatano, D. Clark, and Y. Katoh, “Characterization and qualification of neutron radiation effects–Summary of Japan-USA joint projects for 40 years,” J. Nucl. Mater. 560, 153494 (2022). https://doi.org/10.1016/j.jnucmat.2021.153494
M. Pečovnik, S. Markelj, A. Založnik, and T. Schwarz-Selinger, “Influence of grain size on deuterium transport and retention in self-damaged tungsten,” J. Nucl. Mater. 513, 198–208 (2019). https://doi.org/10.1016/j.jnucmat.2018.10.026
Funding
The work was carried out as part of the state assignment of National Research Center Kurchatov Institute.
Author information
Authors and Affiliations
Corresponding author
Ethics declarations
The authors of this work declare that they have no conflicts of interest.
Additional information
Publisher’s Note.
Pleiades Publishing remains neutral with regard to jurisdictional claims in published maps and institutional affiliations.
Rights and permissions
About this article
Cite this article
Persianova, A.P., Golubeva, A.V. Hydrogen Traps in Tungsten: A Review. Phys. Metals Metallogr. 125, 278–306 (2024). https://doi.org/10.1134/S0031918X23602895
Received:
Revised:
Accepted:
Published:
Issue Date:
DOI: https://doi.org/10.1134/S0031918X23602895